Picture This: Feynman Diagrams
We are born, we travel, we meet, we die. Perhaps you feel this stark biography has left out some important milestones of your life. Still, it nicely sums up the life stories of elementary particles. Feynman diagrams tell those stories. In the process, they transform how physicists think about forces.
I learned of Feynman diagrams when I first encountered Feynman, not in person (unfortunately) but through his memoirs. I was in eleventh grade, a student in Bombay, puttering around my house during a teachersâ strike, when my mother, herself trained as a particle physicist, gave me a copy of Surely Youâre Joking, Mr Feynman!. That book introduced me to the persona of Richard Feynman, the brilliant physicist who cracked jokes and cracked safes and played bongos to boot. Feynmanâs irreverent antics delighted high-school me. But it wasnât until graduate school that I understood the significance of his diagramsâwhat they meant and what they were for.
Feynman diagrams portray the interaction between particles in space and time. Consider the picture above. This particular pair of Feynman diagrams corresponds to a process called Bhabha scattering, the meeting of an electron and a positronâthe electronâs antimatter identical twin. On these diagrams, time runs vertically up, space horizontally. Of course, space is really three-dimensional and our picture has only two dimensionsâone of which weâre already using to represent timeâbut we neednât worry about that. We read these diagrams chronologically, from bottom to top as if we were reading a page from the bottom up, line-by-line, each line a snapshot of a moment in time.
In both diagrams, the electron (labeled e-) comes in from the bottom left, the positron (labeled e+) from the bottom right. Notice that the electron has an arrow pointing up but the positron has an arrow pointing down. Thatâs because, for Feynman, antimatter is secretly matter traveling backwards in time. (Yes, really.) The wiggly lines denote photons, little droplets of light. But these arenât ordinary photons. These are virtual photons, wraith-like and ephemeral, flitting just beneath the threshold of existence. You cannot capture them, you cannot see them. Yet in their brief lives quantum mechanics allows these droplets of unseeable light to do extraordinary things.
Take for instance the diagram on the left. Notice that the photon line runs purely horizontally. Since thereâs no vertical component, itâs all taking place at the same moment in time: the virtual photon essentially travels from one place to another instantaneouslyâlight traveling literally faster than light. The virtual photon connects the electron and positron lines. It thereby transmits momentum and energy between the electron and the positron, which both recoil as a result and scatter.
Next, letâs look at the diagram on the right. As before, the electron comes in (bottom left) and meets the positron (bottom right). But this time the two collide. Notice how the diagram has the electron line going up and bouncing back into the positron line. Feynman gave this bounce-back an astounding interpretation: he suggested that what appears to be two particlesâthe matter electron and the antimatter positronâcan be thought of as just one single particle meeting its own future self going backwards in time. Going further up the diagram, we see that the electron and positron are now gone. Theyâve annihilated each other, leaving behind a wiggly line, which is our old friend, the virtual photon. Finally, some time later, at the topmost junction, the photon vanishes and its energy spontaneously births a new electron-positron pair.
But why are there two diagrams, and not just one? After all, in both diagrams the story starts out the same, with the electron and the positron approaching each other. Yet the narrative unfolds differently, like different choices in a choose-your-own-adventure. So which one really takes place? Well, hereâs the incredible thing. Quantum mechanics says that every imaginable development of the story occurs. Everything that can happen, does happen. There are no roads not taken. The story on the left, with the original electron and positron scattering away, and the story on the right, with the original electron-positron pair replaced by a reincarnated copy, both happen.
Feynman diagrams offer much more than tall tales of particles. To the trained eye, the diagrams are part of a systematic method to calculate the chance of a given interaction in ever-greater detail. There are a set of rulesâcalled Feynman rules of courseâon how to translate a given set of diagrams into an equation representing the likelihood of that interaction happening. For Bhabha scattering, our two Feynman diagrams encode the following equation:
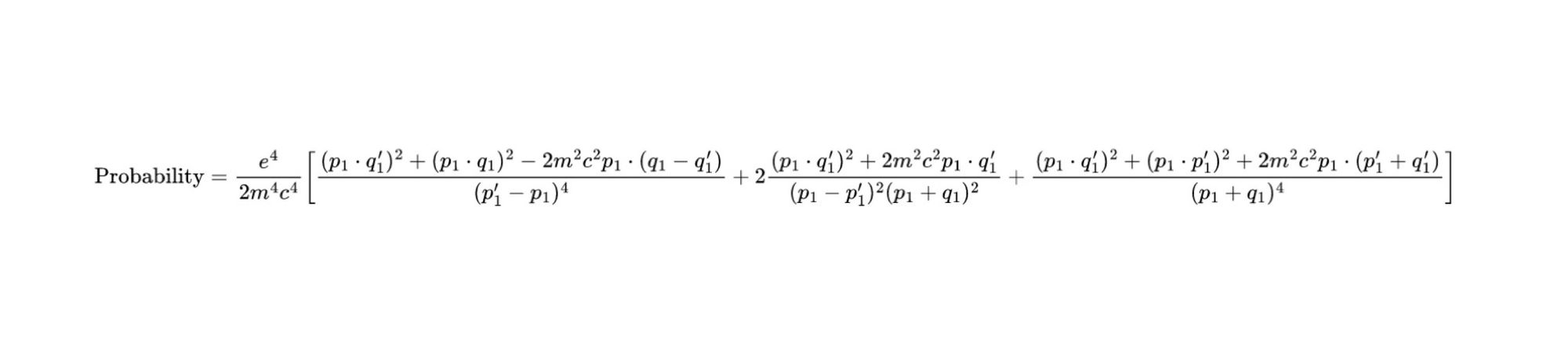
Though seemingly daunting, the calculations are actually much simpler and faster to execute than the abstruse techniques that were developed in the 1940s by Julian Schwinger and others. Using Feynmanâs methods, all you have to do is imagine some particle interaction, sketch its diagrams, apply the Feynman rulesâŠand out pops the likelihood of that interaction. âFeynman brought computation to the masses,â lamented Schwinger, unhappily.
Today, Feynman diagrams are ubiquitous; every graduate student in theoretical physics learns about them, physics departments all over the world have blackboards covered with them. Still, itâs worth mentioning that in recent years physicists have discovered newer techniques, with names like spinor helicity and twistor theory and Wilson loops, that, in certain contexts, do the work of thousands of Feynman diagrams. Weâre still learning about these methods but they hint at the existence of a deeper reality we donât yet fully understand.
More broadly, Feynman diagrams have helped transform how we think of forces. You see, according to quantum mechanics, there exists a duality between waves and particles. Phenomena that we normally call waves (light waves, say, or sound waves) can also be thought of in terms of particles. Similarly, associated with every force field is a type of force-carrying particle: photons for the electromagnetic field, gluons for the nuclear field, hypothetical particles called gravitons for the gravitational field. Feynman diagrams, then, invite us to envision forces in terms of particles.
Take gravity, for instance. There are now (at least) three different ways of thinking about gravity. First, because of Newton, we think of gravity as a force, the mutual attraction between any two objects in the universe: a falling apple and the earth, or the sun and Jupiter. But then, because of Einstein, we see that gravity is, in a sense, not a force at all. Rather, gravity is a manifestation of the curvature of spacetime: gravity is geometry. And now, because of Feynman, we learn that gravity is what arises when two objects exchange virtual gravitons: gravity is transmitted by particles. Incredibly, all of these viewpoints are simultaneously correct. Thatâs theoretical physics for you. âŠ
Subscribe to Broadcast